Solar radiation management
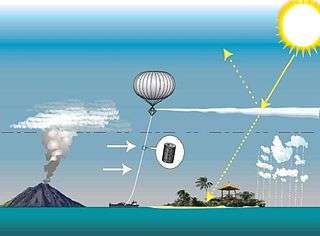
Solar radiation management[1] (SRM) projects (proposed and theoretical) are a type of climate engineering which seek to reflect sunlight and thus reduce global warming.[2] Proposed examples include the creation of stratospheric sulfate aerosols. Their principal advantages as an approach to climate engineering is the speed with which they can be deployed and become fully active, their potential low financial cost, and the reversibility of their direct climatic effects. Solar radiation management projects could, for example, be used as a temporary response while levels of greenhouse gases can be brought under control by greenhouse gas remediation techniques. They would not reduce greenhouse gas concentrations in the atmosphere, and thus do not address problems such as ocean acidification caused by excess carbon dioxide (CO2). By comparison, other climate engineering techniques based on greenhouse gas remediation, such as ocean iron fertilization, need to sequester the anthropogenic carbon excess before any reversal of global warming would occur.
Background
Climate engineering projects have been proposed in order to reduce global warming. The effect of rising greenhouse gas concentrations in the atmosphere on global climate is a warming effect on the planet. By modifying the albedo of the Earth's surface, or by preventing sunlight reaching the Earth by using a solar shade, this warming effect can be cancelled out—although the cancellation is imperfect, with regional discrepancies remaining.[3]
Therefore, solar radiation management, or albedo modification, is considered to be a potential option for addressing climate change. As the National Academy of Sciences states in its 2015 report: "The two main options for responding to the risks of climate change involve mitigation—reducing and eventually eliminating human-caused emissions of CO2 and other greenhouse gases (GHGs)—and adaptation—reducing the vulnerability of human and natural systems to changes in climate. A third potentially viable option, currently under development but not yet widely deployed, is carbon dioxide removal (CDR) from the atmosphere accompanied by reliable sequestration. A fourth, more speculative family of approaches called albedo modification seeks to offset climate warming by greenhouse gases by increasing the amount of sunlight reflected back to space."[4] In this context, solar radiation management is widely viewed as a complement, not a substitute, to climate change mitigation and adaptation efforts. As The Royal Society concluded in its 2009 report: "Geoengineering methods are not a substitute for climate change mitigation, and should only be considered as part of a wider package of options for addressing climate change."[5] Or put another way: "The safest and most predictable method of moderating climate change is to take early and effective action to reduce emissions of greenhouse gases. No geoengineering method can provide an easy or readily acceptable alternative solution to the problem of climate change. Geoengineering methods could, however, potentially be useful in future to augment continuing efforts to mitigate climate change by reducing emissions, and so should be subject to more detailed research and analysis."[5]
The phenomenon of global dimming is widely known, and is not necessarily a climate engineering technique. It already occurs under current conditions, due to aerosols caused by pollution, or caused naturally as a result of volcanoes and major forest fires. However, its deliberate manipulation is a tool of the geoengineer.
By intentionally changing the Earth's albedo, or reflectivity, scientists propose that we could reflect more heat back out into space, or intercept sunlight before it reaches the Earth through a literal shade built in space. A 2% albedo increase would roughly halve the effect of CO2 doubling.[6]
The National Academy of Sciences describes several of the potential benefits and risks of solar radiation management: "Modeling studies have shown that large amounts of cooling, equivalent in scale to the predicted warming due to doubling the CO2 concentration in the atmosphere, can be produced by the introduction of tens of millions of tons of aerosols into the stratosphere. ... Preliminary modeling results suggest that albedo modification may be able to counter many of the damaging effects of high greenhouse gas concentrations on temperature and the hydrological cycle and reduce some impacts to sea ice. Models also strongly suggest that the benefits and risks will not be uniformly distributed around the globe."[7]
The applicability of many techniques listed here has not been comprehensively tested. Even if the effects in computer simulation models or of small-scale interventions are known, there may be cumulative problems such as ozone depletion, which become apparent only from large scale experiments.[8]
Various small-scale experiments have been carried out on techniques such as cloud seeding, increasing the volume of stratospheric sulfate aerosols and implementing cool roof technology.
As early as 1974, Russian expert Mikhail Budyko suggested that if global warming became a problem, we could cool down the planet by burning sulfur in the stratosphere, which would create a haze. The annual cost of delivering a sufficient amount of sulfur to counteract expected greenhouse warming is estimated at 2 to 8 billion USD.[9]
A preliminary study by Edward Teller and others in 1997 presented the pros and cons of various relatively "low-tech" proposals to mitigate global warming through scattering/reflecting sunlight away from the Earth via insertion of various materials in the upper stratosphere, low earth orbit, and L1 locations.[10]
Advantages
Solar radiation management has certain advantages relative to emissions cuts, adaptation, and carbon dioxide removal. Its effect of counteracting climate change would be experienced very rapidly, on the order of months after implementation, whereas the effects of emissions cuts and carbon dioxide removal are delayed because the climate change that they prevent is itself delayed. Some proposed solar radiation management techniques are expected to have very low direct financial costs of implementation. This creates a different problem structure. Whereas the provision of emissions reduction and carbon dioxide removal present collective action problems (because ensuring a lower atmospheric carbon dioxide concentration is a public good), a single countries or a handful of countries could implement solar radiation management. Finally, the direct climatic effects of solar radiation management are reversible on short timescales.
Limitations and risks
As well as the imperfect cancellation of the climatic effect of greenhouse gases,[11] there are other significant problems with solar radiation management as a form of climate engineering.
Incomplete solution to CO2 emissions
Solar radiation management does not remove greenhouse gases from the atmosphere and thus does not reduce other effects from these gases, such as ocean acidification.[12] While not an argument against solar radiation management per se, this is an argument against reliance on climate engineering to the exclusion of greenhouse gas reduction.
Control and predictability
Most of the information on solar radiation management is from models and computer simulations. The actual results may differ from the predicted effect. The full effects of various solar radiation management proposals are not yet well understood.[13] It may be difficult to predict the ultimate effects of projects,[14] with models presently giving varying results.[15] In the cases of systems which involve tipping points, effects may be irreversible. Furthermore, most modeling to date consider the effects of using solar radiation management to fully counteract the increase in global average surface temperature arising from a doubling or a quadrupling of the preindustrial carbon dioxide concentration. Under these assumptions, it overcompensates for the changes in precipitation from climate change. Solar radiation management is more likely to be optimized in a way that balances counteracting changes to temperature and precipitation, to compensate for some portion of climate change, and/or to slow down the rate of climate change.
Side effects
There may be unintended climatic consequences of solar radiation management, such as significant changes to the hydrological cycle[16][17][18] that might not be predicted by the models used to plan them.[14] Such effects may be cumulative or chaotic in nature.[19] Ozone depletion is a risk of techniques involving sulfur delivery into the stratosphere.[20] Not all side effects are negative, and an increase in agricultural productivity has been predicted by some studies due to the combination of more diffuse light and elevated carbon dioxide concentration.[21]
Termination shock
If solar radiation management were masking a significant amount of warming and then were to abruptly stop, the climate would rapidly warm.[22] This would cause a sudden rise in global temperatures towards levels which would have existed without the use of the climate engineering technique. The rapid rise in temperature may lead to more severe consequences than a gradual rise of the same magnitude.
Disagreement
Leaders of countries and other actors may disagree as to whether, how, and to what degree solar radiation would be used. This could exacerbate international tensions.
Weaponization
In 1976, 85 countries signed the U.N. Convention on the Prohibition of Military or Any Other Hostile Use of Environmental Modification Techniques.[23] The Environmental Modification Convention generally prohibits weaponising climate engineering techniques. However, this does not eliminate the risk. If perfected to a degree of controllability and accuracy that is not considered possible at the moment, climate engineering techniques could theoretically be used by militaries to cause droughts or famines.[24] Theoretically they could also be used simply to make battlefield conditions more favourable to one side or the other in a war.[25]
Carnegie's Ken Caldeira said, "It will make it harder to achieve broad consensus on developing and governing these technologies if there is suspicion that gaining military advantage is an underlying motivation for its development..."[26]
Effect on sunlight, sky and clouds
Managing solar radiation using aerosols or cloud cover would involve changing the ratio between direct and indirect solar radiation. This would affect plant life[27] and solar energy.[28] It is believed that there would be a significant effect on the appearance of the sky from stratospheric aerosol injection projects, notably a hazing of blue skies and a change in the appearance of sunsets.[29] Aerosols affect the formation of clouds, especially cirrus clouds.[30]
Roles
SRM has been suggested to control regional climate,[31] but precise control over the geographical boundaries of the effect is not possible.
SRM is temporary in its effect, and thus and long-term restoration of the climate would rely on long-term SRM, unless carbon dioxide removal was subsequently used. However, short-term SRM programs are potentially beneficial.[32]
Forms of proposed solar radiation management
Atmospheric proposals
These projects seek to modify the atmosphere, either by enhancing naturally occurring stratospheric aerosols, or by using artificial techniques such as reflective balloons.
Stratospheric aerosols
Injecting reflective aerosols into the stratosphere is the proposed solar radiation management method that has received the most sustained attention. This technique could give much more than 3.7 W/m2 of globally averaged negative forcing,[33] which is sufficient to entirely offset the warming caused by a doubling of CO2, which is a common benchmark for assessing future climate scenarios. Sulfates are the most commonly proposed aerosols for climate engineering, since there is a good natural analogue with (and evidence from) volcanic eruptions. Explosive volcanic eruptions inject large amounts of sulfur dioxide gas into the stratosphere, which form sulfate aerosol and cool the planet. Alternative materials such as using photophoretic particles, titaniun dioxide, and diamond have been proposed.[34][35][36] Delivery could be achieved using artillery, aircraft (such as the high-flying F15-C) or balloons.[37][38][39] Broadly speaking, stratospheric aerosol injection is seen as a relatively more credible climate engineering technique, although one with potential major risks and challenges for its implementation. Risks include changes in precipitation and, in the case of sulfur, possible ozone depletion.
Marine cloud brightening
Various cloud reflectivity methods have been suggested,[40][41][42] such as that proposed by John Latham and Stephen Salter,[43][44] which works by spraying seawater in the atmosphere to increase the reflectivity of clouds.[45] The extra condensation nuclei created by the spray will change the size distribution of the drops in existing clouds to make them whiter.[46] The sprayers would use fleets of unmanned rotor ships known as Flettner vessels to spray mist created from seawater into the air to thicken clouds and thus reflect more radiation from the Earth.[40][47] The whitening effect is created by using very small cloud condensation nuclei, which whiten the clouds due to the Twomey effect.
This technique can give more than 3.7 W/m2 of globally averaged negative forcing,[33][47] which is sufficient to reverse the warming effect of a doubling of CO2.
Ocean sulfur cycle enhancement
Enhancing the natural marine sulfur cycle by fertilizing a small portion with iron—typically considered to be a greenhouse gas remediation method—may also increase the reflection of sunlight.[48][49] Such fertilization, especially in the Southern Ocean, would enhance dimethyl sulfide production and consequently cloud reflectivity. This could potentially be used as regional solar radiation management, to slow Antarctic ice from melting. Such techniques also tend to sequester carbon, but the enhancement of cloud albedo also appears to be a likely effect.
Terrestrial proposals
Cool roof
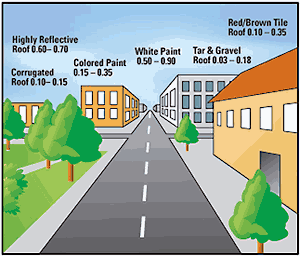
Painting roof materials in white or pale colours to reflect solar radiation, known as 'cool roof' technology, is encouraged by legislation in some areas (notably California).[50] This technique is limited in its ultimate effectiveness by the constrained surface area available for treatment. This technique can give between 0.01-0.19 W/m2 of globally averaged negative forcing, depending on whether cities or all settlements are so treated.[33] This is small relative to the 3.7 W/m2 of positive forcing from a doubling of CO2. Moreover, while in small cases it can be achieved at little or no cost by simply selecting different materials, it can be costly if implemented on a larger scale. A 2009 Royal Society report states that, “the overall cost of a ‘white roof method’ covering an area of 1% of the land surface (about 1012 m2) would be about $300 billion/yr, making this one of the least effective and most expensive methods considered.”[51] However, it can reduce the need for air conditioning, which emits CO2 and contributes to global warming.
Reflective sheeting
Adding reflective plastic sheets covering 67,000 square miles (170,000 km2) of desert every year between 2010 and 2070 to reflect the Sun’s energy.[52][53] may be able give globally averaged 1.74 W/m2 of negative forcing.[33] Although insufficient to fully offset the 3.7 W/m2 of positive forcing from a doubling of CO2, this would still be a significant contribution thereto, and would offset the current level of warming (approx. 1.7 W/m2). However, the effect would be strongly regional, and would not be ideal for controlling Arctic shrinkage, which is one of the most significant problems resulting from global warming. Furthermore, desert albedo modification would be expensive, would compete with other land uses, and would have strongly negative ecological consequences.[5] Finally, the total area required during 2010-70 is larger than all non-polar deserts combined.
Ocean changes
An early geoengineering idea was to use pale coloured floating litter within certain stable oceanic gyres.[54] This litter would tend to group into large and stable areas, such as the Great Pacific Garbage Patch.[55]
Oceanic foams have also been suggested,[56] using microscopic bubbles suspended in the upper layers of the photic zone.
Forestry
Reforestation in tropical areas has a cooling effect. Deforestation of high-latitude and high-altitude forests exposes snow and this increases albedo.[57]
Grassland management
Changes to grassland have been proposed to increase albedo.[58] This technique can give 0.64 W/m2 of globally averaged negative forcing,[33] which is insufficient to offset the 3.7 W/m2 of positive forcing from a doubling of CO2, but could make a minor contribution.
High-albedo crop varieties
Selecting or genetically modifying commercial crops with high albedo has been suggested.[59] This has the advantage of being relatively simple to implement, with farmers simply switching from one variety to another. Temperate areas may experience a 1 °C cooling as a result of this technique.[60] This technique is an example of bio-geoengineering. This technique can give 0.44 W/m2 of globally averaged negative forcing,[33] which is insufficient to offset the 3.7 W/m2 of positive forcing from a doubling of CO2, but could make a minor contribution.
Space-based proposals
Space-based climate engineering projects are seen by many commentators and scientists as being very expensive and technically difficult, with the Royal Society suggesting that "the costs of setting in place such a space-based armada for the relatively short period that SRM geoengineering may be considered applicable (decades rather than centuries) would likely make it uncompetitive with other SRM approaches."[61]
Space mirrors
Mirrors in space: proposed by Roger Angel with the purpose to deflect a percentage of solar sunlight into space, using mirrors orbiting around the Earth.[45][62]
Moon dust
Mining moon dust to create a shielding cloud was proposed by Curtis Struck at Iowa State University in Ames.[63][64][65]
Dispersive solutions
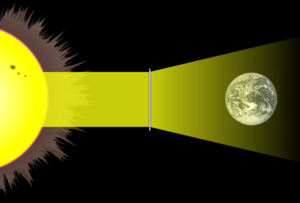
Several authors have proposed dispersing light before it reaches the Earth by putting a very large diffraction grating (thin wire mesh) or lens in space, perhaps at the L1 point between the Earth and the Sun. Using a Fresnel lens in this manner was proposed in 1989 by J. T. Early.[66] Using a diffraction grating was proposed in 1997 by Edward Teller, Lowell Wood, and Roderick Hyde.[10] In 2004, physicist and science fiction author Gregory Benford calculated that a concave rotating Fresnel lens 1000 kilometres across, yet only a few millimeters thick, floating in space at the L1 point, would reduce the solar energy reaching the Earth by approximately 0.5% to 1%. He estimated that this would cost around US$10 billion up front, and another $10 billion in supportive cost during its lifespan.[67] One issue with implementing such a solution is the need to counteract the effects of the solar wind moving such megastructures out of position.
Governance
Climate engineering poses several challenges in the context of governance because of issues of power and jurisdiction.[23] Climate engineering as a climate change solution differs from other mitigation and adaptation strategies. Unlike a carbon trading system that would be focused on participation from multiple parties along with transparency, monitoring measures and compliance procedures; this is not necessarily required by climate engineering. Bengtsson[68] (2006) argues that "the artificial release of sulphate aerosols is a commitment of at least several hundred years". Yet this is true only if a long-term deployment strategy is adopted. Under a short-term, temporary strategy, implementation would instead be limited to decades.[69] Both cases, however, highlight the importance for a political framework that is sustainable enough to contain a multilateral commitment over such a long period and yet is flexible as the techniques innovate through time. There are many controversies surrounding this topic and hence, climate engineering has been made into a very political issue. Most discussions and debates are not about which climate engineering technique is better than the other, or which one is more economically and socially feasible. Discussions are broadly on who will have control over the deployment of climate engineering and under what governance regime the deployment can be monitored and supervised. This is especially important due to the regional variability of the effects of many climate engineering techniques, benefiting some countries while damaging others. The challenge posed by climate engineering is not how to get countries to do it. It is to address the fundamental question of who should decide whether and how climate engineering should be attempted – a problem of governance.[70]
Solar radiation management raises a number of governance challenges. David Keith argues that the cost is within the realm of small countries, large corporations, or even very wealthy individuals.[71] David Victor suggests that climate engineering is within the reach of a lone "Greenfinger," a wealthy individual who takes it upon him or herself to be the "self-appointed protector of the planet".[72][73] However, it has been argued that a rogue state threatening solar radiation management may strengthen action on mitigation.[74]
Legal and regulatory systems may face a significant challenge in effectively regulating solar radiation management in a manner that allows for an acceptable result for society. There are, however, significant incentives for states to cooperate in choosing a specific climate engineering policy, which make unilateral deployment a rather unlikely event.[75]
Some researchers have suggested that building a global agreement on climate engineering deployment will be very difficult, and instead power blocs are likely to emerge.[76]
Public attitudes
There have been a handful of studies into attitudes to and opinions of solar radiation management. These generally find low levels of awareness, uneasiness with the implementation of solar radiation management, cautious support of research, and a preference for greenhouse gas emissions reduction.[77][78] As is often the case with public opinions regarding emerging issues, the responses are highly sensitive to the questions' particular wording and context.
One cited objection to implementing a short-term temperature fix is that there might then be less incentive to reduce carbon dioxide emissions until it caused some other environmental catastrophe, such as a chemical change in ocean water that could be disastrous to ocean life.[79]
See also
References
- ↑ "A Framework to Prevent the Catastrophic Effects of Global Warming using Solar Radiation Management (Geo-Engineering)" (PDF). Thehardlook.typepad.com. Retrieved 2013-10-16.
- ↑ "Metapress Hosting Services for Academic Publishers". Journals.royalsociety.org. Retrieved 2013-10-16.
- ↑ Geoengineering the Climate: Science, Governance, and Uncertainty. London: The Royal Society. September 2009.
- ↑ "Climate Intervention: Reflecting Sunlight to Cool Earth | The National Academies Press". www.nap.edu. Retrieved 2015-09-11.
- 1 2 3 "The Royal Society" (PDF). royalsociety.org. Retrieved 2015-09-11.
- ↑ "The Royal Society" (PDF). royalsociety.org. Retrieved 2015-10-20.
- ↑ "Climate Intervention: Reflecting Sunlight to Cool Earth | The National Academies Press". www.nap.edu. Retrieved 2015-09-14.
- ↑ Mark, Jason (2009). "Hacking the Sky: Geo-Engineering Could Save the Planet... And in the Process Sacrifice the World". Earth Island Journal. San Francisco, CA: Earth Island Institute. 24 (3): 40–46. ISSN 1041-0406. 472240324.
- ↑ McClellan, Justin; Keith, David W.; Apt, Jay (1 January 2012). "Cost analysis of stratospheric albedo modification delivery systems". Environmental Research Letters. p. 034019. doi:10.1088/1748-9326/7/3/034019.
- 1 2 Edward Teller; Roderick Hyde; Lowell Wood (1997). "Global Warming and Ice Ages: Prospects for Physics-Based Modulation of Global Change" (PDF). Lawrence Livermore National Laboratory. Retrieved 21 January 2015. See pages 10-14 in particular.
- ↑ Moreno-Cruz, Juan B.; Ricke, Katharine L.; Keith, David W. (2011). "A simple model to account for regional inequalities in the effectiveness of solar radiation management". Climatic Change. 110 (3–4): 649. doi:10.1007/s10584-011-0103-z.
- ↑ http://infohost.nmt.edu/~chem/wingenter/Wingenter_PeECE_III_GRL_2007.pdf Archived 14 February 2012 at the Wayback Machine.
- ↑ "WWF condemns iron fertilization scheme to fight global warming". News.mongabay.com. December 2012. Retrieved 2012-09-06.
- 1 2 Keith Bower et al., 2006 Computational assessment of a proposed technique for global warming mitigation via albedo-enhancement of marine stratocumulus clouds. Atmos. Res., vol. 82, no. 1-2, 2006, pp. 328–336
- ↑ http://royalsociety.org/page.asp?tip=1&id=6232
- ↑ Bala, G.; Duffy, B.; Taylor, E. (June 2008). "Impact of geoengineering schemes on the global hydrological cycle" (Free full text). Proceedings of the National Academy of Sciences of the United States of America. 105 (22): 7664–7669. Bibcode:2008PNAS..105.7664B. doi:10.1073/pnas.0711648105. ISSN 0027-8424. PMC 2409412
. PMID 18505844.
- ↑ Tilmes, S.; Fasullo, J.; Lamarque, J. F.; Marsh, D. R.; Mills, M.; Alterskjaer, K.; Muri, H.; Kristjánsson, J. N. E.; Boucher, O.; Schulz, M.; Cole, J. N. S.; Curry, C. L.; Jones, A.; Haywood, J.; Irvine, P. J.; Ji, D.; Moore, J. C.; Karam, D. B.; Kravitz, B.; Rasch, P. J.; Singh, B.; Yoon, J. H.; Niemeier, U.; Schmidt, H.; Robock, A.; Yang, S.; Watanabe, S. (2013). "The hydrological impact of geoengineering in the Geoengineering Model Intercomparison Project (GeoMIP)". Journal of Geophysical Research: Atmospheres. 118 (19): 11,036. doi:10.1002/jgrd.50868.
- ↑ "Geoengineering Could Slow Down Global Water Cycle". Sciencedaily.com. 2008-05-28. Retrieved 2013-10-16.
- ↑ Robock, Alan (May–June 2008). "20 reasons why geoengineering may be a bad idea". Bulletin of the Atomic Scientists. 64 (2): 14–18. doi:10.2968/064002006. Retrieved 2009-08-04.
- ↑ The Sensitivity of Polar Ozone Depletion to Proposed Geoengineering Schemes", Science, vol. 320, no. 5880, 30 May 2008, pp. 1201–1204, doi:10.1126/science.1153966
- ↑ Pongratz, J.; Lobell, D. B.; Cao, L.; Caldeira, K. (2012). "Crop yields in a geoengineered climate". Nature Climate Change. 2 (2): 101. Bibcode:2012NatCC...2..101P. doi:10.1038/nclimate1373.
- ↑ Ross, A.; Damon Matthews, H. (2009). "Climate engineering and the risk of rapid climate change". Environmental Research Letters. 4 (4): 045103. Bibcode:2009ERL.....4d5103R. doi:10.1088/1748-9326/4/4/045103.
- 1 2 Robock, A., A. Marquardt, B. Kravitz, and G. Stenchikov (2009). Benefits, Risks, and costs of stratospheric geoengineering, Geophysical Research Letters, 36, D19703, doi:10.1029/2009GL039209
- ↑ "Battlefield Earth". Foreign Policy. Retrieved 2012-09-06.
- ↑ "AF2025 v3c15-1 | Weather as a Force Multiplier: Owning... | (Ch 1)". Fas.org. Retrieved 2012-09-06.
- ↑ Caldiera, Ken; Various (March 18, 2009). "DARPA and Geoengineering". Climate Intervention Google group. Retrieved 2009-03-21.
- ↑ L. Gu et al., "Responses of Net Ecosystem Exchanges of Carbon Dioxide to Changes in Cloudiness: Results from Two North American Deciduous Forests", Journal of Geophysical Research, vol. 104, no. 31, pp. 421–31, 434 (1999); L. Gu et al., "Advantages of Diffuse Radiation for Terrestrial Ecosystem Productivity", Journal of Geophysical Research, vol. 107, (2002); L. Gu et al., "Response of a Deciduous Forest to the Mount Pinatubo Eruption: Enhanced Photosynthesis", Science, vol. 299, pp. 2,035–38 (2003)
- ↑ Balan Govindasamy and Ken Caldeira, "Geoengineering Earth's Radiation Balance to Mitigate CO2-Induced Climate Change", Geophysical Research Letters, vol. 27, pp. 2,141–44 (2000). For the response of solar power systems, see Michael C. MacCracken, "Geoengineering: Worthy of Cautious Evaluation?" Climatic Change, vol. 77, pp. 235–43 (2006)
- ↑ NASA - Geoengineering: Why or Why Not?
- ↑ K. Sassen et al., ";The 5–6 December 1991 FIRE IFO II Jet Stream Cirrus Case Study: Possible Influences of Volcanic Aerosols", Journal of the Atmospheric Sciences, vol. 52, pp. 97–123 (1993)
- ↑ Bernstein, D. N.; Neelin, J. D.; Li, Q. B.; Chen, D. (2013). "Could aerosol emissions be used for regional heat wave mitigation?". Atmospheric Chemistry and Physics. 13 (13): 6373. doi:10.5194/acp-13-6373-2013.
- ↑ Keith, David W.; MacMartin, Douglas G. (2015). "A temporary, moderate and responsive scenario for solar geoengineering". Nature Climate Change. 5 (3): 201. doi:10.1038/nclimate2493.
- 1 2 3 4 5 6 Lenton, T. M., Vaughan, N. E. (2009). "The radiative forcing potential of different climate geoengineering options" (PDF). Atmos. Chem. Phys. Discuss. 9 (1): 2559–2608. doi:10.5194/acpd-9-2559-2009.
- ↑ Keith, D. W. (2010). "Photophoretic levitation of engineered aerosols for geoengineering". Proceedings of the National Academy of Sciences. 107 (38): 16428–16431. doi:10.1073/pnas.1009519107. PMC 2944714
. PMID 20823254.
- ↑ Weisenstein, D. K.; Keith, D. W. (2015). "Solar geoengineering using solid aerosol in the stratosphere". Atmospheric Chemistry and Physics Discussions. 15 (8): 11799–11851. doi:10.5194/acpd-15-11799-2015.
- ↑ Ferraro, A. J., A. J. Charlton-Perez, E. J. Highwood (2015). "Stratospheric dynamics and midlatitude jets under geoengineering with space mirrors and sulfate and titania aerosols". Journal of Geophysical Research: Atmospheres. 120 (2): 414–429. doi:10.1002/2014JD022734. Retrieved 8 June 2015.
- ↑ Crutzen, P. J. (2006). "Albedo Enhancement by Stratospheric Sulfur Injections: A Contribution to Resolve a Policy Dilemma?" (PDF). Climatic Change. 77 (3–4): 211–220. doi:10.1007/s10584-006-9101-y.
- ↑ Davidson, P.; Burgoyne, C.; Hunt, H.; Causier, M. (2012). "Lifting options for stratospheric aerosol geoengineering: Advantages of tethered balloon systems". Philosophical Transactions of the Royal Society A: Mathematical, Physical and Engineering Sciences. 370 (1974): 4263. Bibcode:2012RSPTA.370.4263D. doi:10.1098/rsta.2011.0639.
- ↑ "Can a Million Tons of Sulfur Dioxide Combat Climate Change?". Wired.com. 23 June 2008. Retrieved 2013-10-16.
- 1 2 Latham, J. (1990). "Control of global warming" (PDF). Nature. 347 (6291): 339–340. Bibcode:1990Natur.347..339L. doi:10.1038/347339b0.
- ↑ Latham, J.; Salter, S. "Preventing global warming by increasing cloud albedo" (PDF). Retrieved 20 April 2008. (A brief handout, with artist's renderings.)
- ↑ Keith Bower; et al. (2006). "Assessment of a Proposed Technique for Global Warming Mitigation via Albedo-Enhancement of Marine Stratocumulus Clouds". Atmospheric Research. 82 (1–2): 328–336. Bibcode:2006AtmRe..82..328B. doi:10.1016/j.atmosres.2005.11.013.
- ↑ Latham, J. (2002). "Amelioration of global warming by controlled enhancement of the albedo and longevity of low-level maritime clouds" (PDF). Atmos. Sci. Lett. 3 (2–4): 52–58. Bibcode:2002AtScL...3...52L. doi:10.1006/asle.2002.0099.
- ↑ Salter, S, G. Sortino & J. Latham (2008). "Sea-going hardware for the cloud albedo method of reversing global warming". Phil. Trans. R. Soc. A. 366 (1882): 3989–4006. Bibcode:2008RSPTA.366.3989S. doi:10.1098/rsta.2008.0136. PMID 18757273.
- 1 2 "Programmes | Five Ways To Save The World". BBC News. 2007-02-20. Retrieved 2013-10-16.
- ↑ Panel on Policy Implications of Greenhouse Warming, National Academy of Sciences, National Academy of Engineering, Institute of Medicine (1992). Policy Implications of Greenhouse Warming: Mitigation, Adaptation, and the Science Base. The National Academies Press. ISBN 0-585-03095-2.
- 1 2 Latham, J., P.J. Rasch, C.C.Chen, L. Kettles, A. Gadian, A. Gettelman, H. Morrison, K. Bower, T.W.Choularton (2008). "Global Temperature Stabilization via Controlled Albedo Enhancement of Low-level Maritime Clouds". Phil. Trans. Roy. Soc. A. 366 (1882): 3969–87. Bibcode:2008RSPTA.366.3969L. doi:10.1098/rsta.2008.0137. PMID 18757272.
- ↑ Wingenter, Oliver W.; Haase, Karl B.; Strutton, Peter; Friederich, Gernot; Meinardi, Simone; Blake, Donald R.; Rowland, F. Sherwood (2004-06-08). "Changing concentrations of CO, CH4, C5H8, CH3Br, CH3I, and dimethyl sulfide during the Southern Ocean Iron Enrichment Experiments". Proceedings of the National Academy of Sciences of the United States of America. 101 (23): 8537–8541. doi:10.1073/pnas.0402744101. ISSN 0027-8424. PMC 423229
. PMID 15173582.
- ↑ Wingenter, Oliver W.; Elliot, Scott M.; Blake, Donald R. (November 2007). "New Directions: Enhancing the natural sulfur cycle to slow global warming". Atmospheric Environment. 41 (34): 7373–5. doi:10.1016/j.atmosenv.2007.07.021.
- ↑ Hashem Akbari; et al. (2008). "Global Cooling: Increasing World-wide Urban Albedos to Offset CO2" (PDF).
- ↑ "The Royal Society" (PDF). royalsociety.org. Retrieved 2015-11-09.
- ↑ Alvia Gaskill. "Desert Area Coverage". Global Albedo Enhancement Project.
- ↑ "Global Albedo Enhancement Project: Surface Albedo Enhancement Principle". Global-warming-geo-engineering.org. Retrieved 2013-10-16.
- ↑ "Solar Radiation Management (GeoEngineering)". Retrieved 23 April 2011.
- ↑ "What is the Great Pacific Garbage Patch?". 21st Century Challenges. Retrieved 23 April 2011.
- ↑ Evans, J.; Stride, E.; Edirisinghe, M.; Andrews, D.; Simons, R. (2010). "Can oceanic foams limit global warming?". Climate Research. 42 (2): 155–160. doi:10.3354/cr00885.
- ↑ Archived 21 November 2008 at the Wayback Machine.
- ↑ Hamwey, Robert M. (2005). "Active Amplification of the Terrestrial Albedo to Mitigate Climate Change: An Exploratory Study". Mitigation and Adaptation Strategies for Global Change. 12 (4): 419. arXiv:physics/0512170
. doi:10.1007/s11027-005-9024-3.
- ↑ "A high-albedo diet will chill the planet - environment - 15 January 2009". New Scientist. Retrieved 2013-10-16.
- ↑ Ridgwell, A; Singarayer, J; Hetherington, A; Valdes, P (2009). "Tackling Regional Climate Change By Leaf Albedo Bio-geoengineering". Current Biology. 19 (2): 146–50. doi:10.1016/j.cub.2008.12.025. PMID 19147356.
- ↑ "The Royal Society" (PDF). royalsociety.org. Retrieved 2015-11-18.
- ↑ David W. Keith (2000). "Geoengineering the climate: History and Prospect". Annual Review of Energy and the Environment. 25 (1): 245–284. doi:10.1146/annurev.energy.25.1.245.
- ↑ Journal of the British Interplanetary Society, vol 60, p 1
- ↑ Roger Angel; S. Pete Worden (Summer 2006). "Making Sun-Shades from Moon Dust". National Space Society, Ad Astra. 18 (1).
- ↑ "Space Ring Could Shade Earth and Stop Global Warming". LiveScience. 2005-06-27. Retrieved 2013-10-16.
- ↑ J. T. Early (1989). "Space-Based Solar Shield To Offset Greenhouse Effect". Journal of the British Interplanetary Society. 42. pp. 567–569. This proposal is also discussed in footnote 23 of Edward Teller; Roderick Hyde; Lowell Wood (1997). "Global Warming and Ice Ages: Prospects for Physics-Based Modulation of Global Change" (PDF). Lawrence Livermore National Laboratory. Retrieved 21 January 2015.
- ↑ See Russell Dovey, "Supervillainy: Astroengineering Global Warming and Bill Christensen, "Reduce Global Warming by Blocking Sunlight".
- ↑ Bengtsson, L. (2006) 'Geo-engineering to confine climate change: is it at all feasible?' Climatic Change 77: 229–234
- ↑ http://www.nature.com/nclimate/journal/v5/n3/full/nclimate2493.html
- ↑ Barrett, S (2007) Why cooperate? The incentive to supply global public goods. Oxford University Press, Oxford
- ↑ David Keith. "Engineering the Planet" (PDF). pp. "3–4,8". Retrieved 2008-04-08.
- ↑ David G. Victor (2008). "On the regulation of geoengineering". Oxford Review of Economic Policy. Oxford University Press. 24 (2): 322–336. doi:10.1093/oxrep/grn018.
- ↑ "The Geoengineering Option". Foreign Affairs. Retrieved 2015-11-18.
- ↑ Millard-Ball, A. (2011). "The Tuvalu Syndrome". Climatic Change. 110 (3–4): 1047–1066. doi:10.1007/s10584-011-0102-0.
- ↑ Joshua Horton (2011). "Geoengineering and the myth of unilateralism: pressures and prospects for international cooperation". Stanford J Law Sci Policy (2): 56–69.
- ↑ Ricke, K. L.; Moreno-Cruz, J. B.; Caldeira, K. (2013). "Strategic incentives for climate geoengineering coalitions to exclude broad participation". Environmental Research Letters. 8: 014021. Bibcode:2013ERL.....8a4021R. doi:10.1088/1748-9326/8/1/014021.
- ↑ Mercer, A. M.; Keith, D. W.; Sharp, J. D. (2011). "Public understanding of solar radiation management". Environmental Research Letters. 6 (4): 044006. doi:10.1088/1748-9326/6/4/044006.
- ↑ Merk, Christine; Pönitzsch, Gert; Kniebes, Carola; Rehdanz, Katrin; Schmidt, Ulrich (2015-02-10). "Exploring public perceptions of stratospheric sulfate injection". Climatic Change. 130 (2): 299–312. doi:10.1007/s10584-014-1317-7. ISSN 0165-0009.
- ↑ Gregory Benford (Comments at the 64th World Science Fiction Convention, August 2006.)
Further reading
- Granger Morgan, Katharine Ricke (2010). An Opinion Piece for the International Risk Governance Council. Cooling the Earth Through Solar Radiation Management: The need for research and an approach to its governance. ISBN 978-2-9700672-8-3
- National Research Council. Climate Intervention: Reflecting Sunlight to Cool Earth. Washington, DC: The National Academies Press, 2015. ISBNs: Prepublication: 978-0-309-36821-6; Paperback (forthcoming): 978-0-309-31482-4