Diet-induced obese
The Diet-Induced Obesity model (DIO model) is an animal model that studies obesity, caused by being fed high-fat and/or high-density diets, imitating the food we see so readily available in society today.[1] Typically mice, rats, dogs or non-human primates are used in these models. These animals can then be used to study in vivo obesity, obesity's comorbidities, and other related diseases. Users of such models must take into account the duration and type of diet (e.g. hydrated gels vs. dry pellets) as well as the environmental conditions and age of the animals, as each may promote different bodyweights, fat percentages, or behaviors.[2]
Driven by the worldwide epidemic of obesity, particularly in the Western world, the DIO model has been integral in understanding the relationship between high-fat/high-density diets and obesity, including the discovery of Akt and mTOR, signals in the body linked to obesity and insulin resistance.[3] However, while many insights into the control of obesity have come from experiments since its introduction in 1949, the use of animal models does restrict our ability to extrapolate findings to humans.[4]
History of the Model
The DIO model was developed in response to growing concerns over the health effects of obesity, as well as the rapid growth of the obesity rate in countries around the world. As such, the model was developed in order to create a controlled environment for the study of how obesity develops, as well as its effects. The model was used as early as 1949, and has expanded far and wide in its use and goals.[5]
Date | Event |
---|---|
September 1978 | First study tested to find correlation between obesity and palatable foods high in fats and lipids, but no conclusion could be reached.[6] |
1982 | Study testing the effects of obesity on the sympathetic nervous system of rats, namely blood pressure. It was found that obesity had a stimulating effect, increasing blood pressure and increasing the risk of hypertension.[7] |
1995 | A study conducted in order to study the expression of the ob gene in rat adipose tissue through the control of their diets. The study also checked for the effects of diabetes on the gene expression.[8] |
June 2002 | Göttingen minipig "pilot" study: published paper on a three month long experiment testing a nonrodent model to see if results could be accepted in DIO research or be compared to results of those studies where rodents were tested.[9] |
2007 | Continued exploration of diet-induced obesity led to a study of rats as a potential model subject. This genomic based study induced obesity in rats and subsequently analyzed RNA microarrays to characterize the rats metabolic response and resultant insulin sensitivity.[1] |
2009 | The model is used to challenge beliefs about the causes of obesity in the population, as a group of scientists decided to test the notion that obesity is a result of overnutrition and could be controlled by limiting meal sizes.[10] |
2010 | Continuing the trend of the model's usage in determining causes of obesity, a group of researchers notice the changes across the diets of several Americans and conduct an experiment to understand why obesity was up when fat consumption was down. For this, they investigate the connection between the types of fat, meal timings and size, and weight gain as well as the reversibility of diet-induced obesity.[11] |
July 2011 | Different Diet Stimuli: Diets varying from hyperlipic, hypercaloric, cholesterol-rich, and cafeteria diets were tested on rodents to see which had the most impact on body size and to determine differences between metabolic responses in rodents and humans.[12] |
2012 | A 2012 study further explored the viability of the diet-induced obesity model by testing several mice for their reactivities to a high-caloric diet. The resulting data found some mice (the B6 mouse) responded to diet-induced obesity most similarly to humans with regards to several parameters including fat content, relative organ size, and general body composition.[3] |
Other Causes of Obesity
Social Determinants
Social and environmental determinants may also induce the onset of obesity. Social class may affect individual access to proper nutritional education and may hinder an individual's ability to make healthy lifestyle choices. Additionally, samples of low-income women and children were also shown to have higher rates of obesity because of stress. Exposure to pollutants such as smoke and second-hand smoke have also shown direct correlations to obesity.
Gut bacteria
Studies on the relationship between infectious agents and weight gain show that certain species of gut flora can affect metabolic processes. This correlation links these gut bacteria to an inability to digest complex polysaccharides. Certain viruses, specifically the AD-36 adenovirus, have been shown to increase body fat in laboratory animals.
Sedentary lifestyle
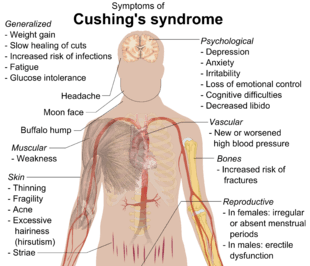
Living a sedentary lifestyle is one of the leading factors in causing obesity.[3] As of 2016, over 30% of people in the world don't get enough exercise.[13]
Genetic-based obesity
Genetic mutations to genes monitoring metabolism and appetite predispose people to obesity. Various syndromes resulting in genetic polymorphisms lead to obesity.[14] A few common examples are: Prader-Willi syndrome, Bardet-Biedl syndrome, Cohen syndrome, and MOMO syndrome.
Other illnesses
Multiple mental and physical illnesses, along with some of the medications that treat such illnesses can increase someone's risk of obesity.[15] Some examples of other illnesses are hypothyroidism, Cushing's syndrome, and growth hormone deficiency.
Limitations

Obesity is affected by "environmental, biological, and psychosocial pressures",[17] therefore it is understandable that several limitations are established when translating results between the results of a diet induced obesity model in a lab and humans. While models are an important method of investigating the influences of obesity and drug testing, it is important to understand the limits of the model's overall ability to resemble the human obesogenic pathophysiology.[18] Such limitations can be divided into three broad categories—biological, dietary and experimental differences—factors including, but not limited to, the genetic makeup of the species or strain, the environment in which the specimen is held (temperature, light, number of animals), age, sex, the duration of the experiment, and the texture or type of rations fed to the animals.[2]
Biological
Numerous sources of biological variation arise in rodents before translating results to humans is even considered. For instance, the age at which mice begin the high-fat diet greatly impacts the metabolic effects.[19] In the strain of mice most commonly used for DIO models, C57BL/6J, mice who started the diet at 10 weeks old showed lower increases in body weight and cholesterol than mice who started at 54 weeks, despite the same diet type and duration.[20] Similarly, 6-week old mice did not develop type diabetes, while 7-8-month old mice did become diabetic due to differences in β-cell activity.[21]
Furthermore, the strain and sex of the rodent impacts the response to the model. Some common mouse strains show large variations in their level of resistance to obesity.[22] Further variation is seen when the sex is also factored in; males of the S5B/P1 strain showed 12% weight gain, while females gained no weight at all.[23] Even within a single strain, large amounts of variation in the phenotype can be seen, despite each mouse having identical genetic backgrounds, which greatly hinders reproducibility.[24]
This has led to cases of studies that used the same strain of mice concluding that the strain is prone to becoming obese in one study and resistant in the other study.[25][26] So, despite the fact that variability is clearly present in humans, variability in mice is once again detrimental to the reproducibility of results obtained from the diet induced obesity model.[24]
When functional genomics is applied, few commonalities between the gene expression of DIO vs control rodents and obese vs non-obese humans are found.[1][24] This is particularly true in the case of glucose regulation, which greatly hinders the ability to apply the results of the DIO model to humans, especially for drug development.[1][27]
Dietary
In terms of dietary, there are numbers of "high fat diet-HFD" experiments that have been done in rodents realizing the difficulties in interpreting the literature composition of high fat diet into actual experiment.[28]
With varied sources and types of fat, researches have shown the complexity of defining a model of a high fat diet that can both resemble human meals and accurately quantify the nutrient contents. In fact, recent studies prefer to use purified ingredients to exams the effect of each dietary on rodents’ metabolism and their phenotype.[29]
There is significant variation in the results appearing in either experimental rodents that were fed with high fat diets made up from different ingredients and from purified ingredient. Moreover, the sources of fat were diverse from butter, beef tallow, and lard, to vegetable and fish oils.[18] The weight gaining effects on mice when feeding those with high beef fat diet is 1.38 times more remarkable than feeding them with canola oil.[18]
Additionally, they both can be found in rodents’ and humans’ diets. Researchers have created the study models of high carbohydrate and high proteins. However, the variation in the results of those models has caused the difficulties to interpret and find the relation to human case. According to a review on Nutrition & Diet, there were number of studies ignoring the nutrient composition differences between the control and the high fat diets, but comparing the phenotype expression of these two groups to conclude the influence of hypercaloric diets in causing obesity.[18]
Similar to fat (lipid), the sources of protein and carbohydrate are also essential contributors to the outcomes of high fat diets and control diets rodents group. For instance, casein causes more weight gaining compared to soy.[18] Moreover, different mouse strains can express contradicting results, even though they are both fed with same protein and carbohydrate ratios.[18] In addition to protein, Fructose, a carbohydrate, has impact on fat deposition, plasma insulin, leptin, thyroid, estradiol, and corticosterone levels, lipogenesis, and lipolysis in the adipose tissue of the rat.[18] "Glucose-sweetened beverages," however, did not caused as significant influence as "fructose-sweetened beverages" in promoting visceral adipose, gaining weight, interrupting lipid syntheses, and damaging lipoprotien rebuilding process.[18]
Given the diversity in human food and each human individually distinguished metabolic capacity, the results of testing the diet induce obesity in rodents are limited in term of translatability. Furthermore, dietary components would provide a spectrum of results since both type of diets one with mixture of ingredients-"cafeteria diet" and one with predefined ingredients would alter different impact on the metabolism of the body.[18]
Experimental
Intra and Inter Laboratories results can differ due to variation in the experimental factors such as the protocol used, housing, temperature, light/dark cycle and the duration of study.[18]
Laboratories temperature which are usually (18-22 °C) are lower than the thermoneutrality of the model organism like mice which are about 30 °C. That can introduce hyperphagia in the organism in effort of increasing their metabolism to generate heat energy for the body. This level of thermal stress can also have unaccounted effects on the organism's other metabolic processes.[18]
Furthermore, the spatial confinements in housing organisms can affect the brain chemistry in social creatures like mice and rats, making them more vulnerable to slower brain development and abnormalities due to the lack of social interactions.[32] The psychological effects can introduce further discrepancy in the studies that makes it difficult to render proper data and further hinders the possibility of recapitulating such experiments in humans.
Rodents are nocturnal and are mostly feeding at night, in their natural habitat. The changes in the light/dark cycle of laboratories can alter their circadian rhythm which can affect their metabolism. Besides that several studies used genetically modified mouse models that have decreased circadian rhythmicity gene. These cases have resulted in the increase of metabolic syndrome in the mouse models as well as resulted in obesity.[33] The change in the phenotype can be a result of genetic modification of circadian clock gene, HFD, disruption of the circadian cycle by changes in light and dark cycle or can be due to the combination of all the factors.[33] Further research is required to find the exact cause.
The cause of Diabetes mellitus type 2 (T2D) in humans are far more complicated than the sole consumption of High Fat Diet (HFD). The mental, emotional and cultural factors along with insulin resistance and hyperphagia are known to increase the occurrence of T2D in humans.[18] However, T2D in model organisms are introduced via surgery of partial/ whole pancreas, or using chemicals such as Streptozotocin. Streptozotocin inhibits the ability of pancreatic β cells to produce insulin, and depending on the dosage used, the result can be partial or absolute inhibition. It can also interfere with other cell signallingpathways as well as affects the content of certain isozymes in organs like liver, brain and kidneys.[34] The lack of proper representation of the undertaking of the experiment can introduce T2D, but fails to reflect the mechanism through which these disease takes place in humans.
Model Species
Mice
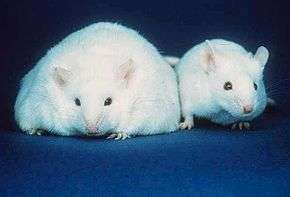
Mice are used by scientists as diet-induced obesity models in experiments because they have mammalian physiological systems similar to those in humans. They also can be bred or genetically engineered to be resistant to certain diseases, which can be important for studies of these diseases and/or their influence on other biological systems.[35]
Scientists used mice to study the effect of lymphotoxins on metabolism. Mice without lymphotoxin alpha, lymphotoxin beta, or a lymphotoxin beta receptor had poorly composed microbiota, which made them resistant to obesity. Mice without lymphotoxin alpha, lymphotoxin beta, or a lymphotoxin beta receptor gained less weight on a high-fat diet than wild type mice did, even after remaining on a high-fat diet for a prolonged period of time.[36]Mice are used to study the significance of certain chemicals on obesity. For example, mice were put on a high-fat diet, but given either tap water, green tea, or Goishi tea to drink. The mice who drank Goishi tea gained less weight and had less sugar in their blood than the mice who drank tap water and green tea. The researchers found that Goishi tea prevented the growth of adipocytes and prevented changes caused by tumor necrosis factor alpha and interleukin 6 when the mice were on a high fat diet.[37] Another chemical studied to find an effect on obesity was propolis. To study the effects of the fungus, scientists injected it into mice while they were on an unrestricted high-fat diet. The researchers found that the mice injected with propolis had less adipose tissue, glucose, and cholesterol than the mice who were not administered propolis. Similar effects were seen in mice who were slowly introduced to propolis while on the high-fat diet.[38]
Some species of mice are used in research because they have specific traits important for a study rather than for similarity to humans. For example, Apodemus chevrieri is used in studies of metabolism because the length of the day determines their metabolism instead of their diet. In studies with A. chevrieri, scientists found that even if metabolism is controlled by day length, the mice could still gain weight with a high-fat diet.[39]
Rats
Rats have also been used in the diet-induced obesity model. Commonly used in medical research, rats were specifically chosen to study the diet-induced obesity model because of the features that they share with humans. One such characteristic is insulin resistance, which comes with diet-induced obesity in both rats and humans. Additionally, obesity remains in both species for long periods of time after it has initially set in. Because of the commonalities between the two, rats may prove to be helpful in the search for the cause of human obesity.[40] For example, in one experiment, male Sprague-Dawley rats were given either a low fat or a high fat diet, with the high fat diet containing 35% more fat than the low fat diet. The results of the study illustrate that the high fat diet rats had a higher adiposity index than the low fat diet rats.[41]
Dogs
Dogs are used for research because they can be domesticated, and because they have been used in studies concerning diabetes in the past.[42] For example, dogs were used as subjects in a study of the effects of diet-induced obesity on insulin dispersion.[43] In this experiment, it was found that a high-fat diet caused insulin resistance, contributing to cardiovascular disease, cancer, and type 2 diabetes.
Research done with model


Methodology
General methodology
As in most science experiments, a good experiment using the diet-induced obesity model has an experimental and a control group. The control group is given a diet with low percentage of total energy from fat (e.g. 10%), while the experimental group is given a diet with a high percentage of total energy from fat (e.g. 60%).[3] The effect of the diet is quantified using the measures detailed below. Often, the experiment aims to see how obesity affects some other physiological or behavioral outcome, so other measures may be taken. Common such measures include stress (both physiological and psychological), changes in hormones, and insulin.[45]
Measures of obesity
The outcome measure of obesity is usually either the gain of body weight or body fat. The body weight gain is quantified using the difference in the raw mass of the animal or in the Lee index (an index similar to the BMI in humans). The body fat gain is quantified either indirectly through the weight gain, or directly using dual-energy X-ray absorptiometry.[45] When studying effects of obesity on diabetes, a fasting blood sugar test is also done before and after the diet.[3]
Diets
Scientists have successfully induced obesity in animals using a wide range of diets. Although generally diets containing more than 30% of total energy from fat are considered to induce obesity, scientists have induced obesity with diets containing 13% to 85% of total energy from fat. The specific fatty foods used in the diets vary across studies, ranging from Crisco to lard to palm oil.[45]
Behavioral changes
Sensory stimulation from high-fat foods is one behavioral mechanism in the diet-induced obesity model—humans’ and rats’ neural proclivity for the texture, smell, and taste of high-fat foods stimulates "selection, consumption, digestion and absorption"[5] of those foods. According to some studies, time, frequency, and quantity of feedings are other behavioral factors in the DIO model. Some research shows that nightly eating, low eating frequency, and large meal size may contribute to diet-induced obesity. Depression and long-term stress are also reported mechanisms contributing to obesity via increased food intake.[5]
Physiological changes
Weight gain is the primary effect of diet-induced obesity, but there are a variety of additional physiological side effects. One such side effect is that the body gains more fat cells. The increased number of fat cells persists even after the diet becomes lower in fat. Weight put on during the high-fat diet also tends to persist.[44] Changes in the composition of the body are accompanied by hormonal changes. High levels of leptin and insulin are produced; at the same time, the body becomes resistant to both. Insulin resistance in particular is fed by the addition of more fat cells.[45]
See also
References
- 1 2 3 4 Li, Shuyu; Zhang, Hong-Yan; Hu, Charlie C.; Lawrence, Frank; Gallagher, Kelly E.; Surapaneni, Anupama; Estrem, Shawn T.; Calley, John N.; Varga, Gabor (2008-04-01). "Assessment of Diet-induced Obese Rats as an Obesity Model by Comparative Functional Genomics". Obesity. 16 (4): 811–818. doi:10.1038/oby.2007.116. ISSN 1930-739X.
- 1 2 3 Reuter, Tanja Y. (2007-01-01). "Diet-induced models for obesity and type 2 diabetes". Drug Discovery Today: Disease Models. Metabolic disorders. 4 (1): 3–8. doi:10.1016/j.ddmod.2007.09.004.
- 1 2 3 4 5 6 Wang, Chao-Yung; Liao, James K. (2012-01-01). "A Mouse Model of Diet-Induced Obesity and Insulin Resistance". Methods in molecular biology (Clifton, N.J.). 821: 421–433. doi:10.1007/978-1-61779-430-8_27. ISSN 1064-3745. PMC 3807094
. PMID 22125082.
- ↑ Template:Cite jDiet-induced models for obesity and type 2 diabetesournal
- 1 2 3 "High-fat diet-induced obesity in animal models". Nutrition Research Reviews. 23: 270–299. 2010.
- ↑ Faust, I. M., Johnson, P. R., Stern, J. S., & Hirsch, J. (1978). Diet-induced adipocyte number increase in adult rats: a new model of obesity. The American journal of physiology, 3, E279-86.
- ↑ Young, James B.; Landsberg, Lewis (1982-01-01). "Diet-induced changes in sympathetic nervous system activity: Possible implications for obesity and hypertension". Journal of Chronic Diseases. Hypertension and Obesity: Epidemiologic, Physiologic and therapeutic considerations. 35 (12): 879–886. doi:10.1016/0021-9681(82)90118-7.
- ↑ Becker, Dominique J.; Ongemba, Lumbe N.; Brichard, Vincent; Henquin, Jean-Claude; Brichard, Sonia M. (1995-09-11). "Diet- and diabetes-induced changes of ob gene expression in rat adipose tissue". FEBS Letters. 371 (3): 324–328. doi:10.1016/0014-5793(95)00943-4.
- ↑ Olholm Larsen, Marriane; Rolin, Bidda; Wilkin, Michael; Carr, Richard David; Svendsen, Ove (June 2002). "High-Fat High-Energy Feeding Impairs Fasting Glucose and Increases Fasting Insulin Levels in the Göttingen Minipig". Annals of the New York Academy of Science. 967: 414–423. doi:10.1111/j.1749-6632.2002.tb04297.x.
- ↑ Furnes, M. W., Zhao, C. M., & Chen, D. (2009). Development of obesity is associated with increased calories per meal rather than per day. A study of high-fat diet-induced obesity in young rats. Obesity surgery, 10, 1430–1438.
- ↑ Niloofar, Hariri; Thibault, Louise. "High-fat diet-induced obesity in animal models". Nutrition Research Reviews. 23.
- ↑ Campos Rosini, Tiago; Sanchez Ramos de Silva, Adelino; de Moraes, Camila (February 10, 2012). "Diet-induced obesity: rodent model for the study of obesity-related disorders". ScienceDirect. 58 (3).
- ↑ "WHO | Physical Inactivity: A Global Public Health Problem". World Health Organization. Retrieved November 9, 2016.
- ↑ Poirier P, Giles TD, Bray GA, Hong Y, Stern JS, Pi-Sunyer FX, Eckel RH (May 2006). "Obesity and cardiovascular disease: pathophysiology, evaluation, and effect of weight loss". Arterioscler. Thromb. Vasc. Biol.(Review). 26 (5): 968–76. doi:10.1161/01.ATV.0000216787.85457.f3. PMID 16627822.
- ↑ Rosén T, Bosaeus I, Tölli J, Lindstedt G, Bengtsson BA (1993). "Increased body fat mass and decreased extracellular fluid volume in adults with growth hormone deficiency". Clin. Endocrinol. (Oxf). 38 (1): 63–71. doi:10.1111/j.1365-2265.1993.tb00974.x. PMID 8435887.
- ↑ "Nutrition & Diabetes - Figure 1 for article: You are what you eat, or are you[quest] The challenges of translating high-fat-fed rodents to human obesity and diabetes". www.nature.com. Retrieved 2016-11-10.
- ↑ Giles, Erin D.; Jackman, Matthew R.; MacLean, Paul S. (2016-01-01). "Modeling Diet-Induced Obesity with Obesity-Prone Rats: Implications for Studies in Females.". Clinical Nutrition. 3: 50. doi:10.3389/fnut.2016.00050.
- 1 2 3 4 5 6 7 8 9 10 11 12 Lai, M.; Chandrasekera, P. C.; Barnard, N. D. (2014-09-08). "You are what you eat, or are you? The challenges of translating high-fat-fed rodents to human obesity and diabetes". Nutrition & Diabetes. 4 (9): e135. doi:10.1038/nutd.2014.30. PMC 4183971
. PMID 25198237.
- ↑ de Castro, Uberdan Guilherme Mendes; dos Santos, Robson Augusto Souza Augusto Souza; Silva, Marcelo Eustáquio; de Lima, Wanderson Geraldo; Campagnole-Santos, Maria José; Alzamora, Andréia Carvalho (2013-01-01). "Age-dependent effect of high-fructose and high-fat diets on lipid metabolism and lipid accumulation in liver and kidney of rats". Lipids in Health and Disease. 12: 136. doi:10.1186/1476-511X-12-136. ISSN 1476-511X. PMC 3849586
. PMID 24044579.
- ↑ Korou, Laskarina-Maria A.; Doulamis, Ilias P.; Tzanetakou, Irene P.; Mikhailidis, Dimitri P.; Perrea, Despina N. (2013-10-01). "The effect of biological age on the metabolic responsiveness of mice fed a high-fat diet". Laboratory Animals. 47 (4): 241–244. doi:10.1177/0023677213480768. ISSN 0023-6772. PMID 23760563.
- ↑ Tschen, Shuen-Ing; Dhawan, Sangeeta; Gurlo, Tatyana; Bhushan, Anil (2009-06-01). "Age-Dependent Decline in β-Cell Proliferation Restricts the Capacity of β-Cell Regeneration in Mice". Diabetes. 58 (6): 1312–1320. doi:10.2337/db08-1651. ISSN 0012-1797. PMC 2682690
. PMID 19228811.
- ↑ West, D. B.; Boozer, C. N.; Moody, D. L.; Atkinson, R. L. (1992-06-01). "Dietary obesity in nine inbred mouse strains". The American Journal of Physiology. 262 (6 Pt 2): R1025–1032. ISSN 0002-9513. PMID 1621856.
- ↑ Schemmel, R.; Mickelsen, O.; Gill, J. L. (1970-09-01). "Dietary obesity in rats: Body weight and body fat accretion in seven strains of rats". The Journal of Nutrition. 100 (9): 1041–1048. ISSN 0022-3166. PMID 5456549.
- 1 2 3 Lai, M.; Chandrasekera, P. C.; Barnard, N. D. (2014-09-08). "You are what you eat, or are you? The challenges of translating high-fat-fed rodents to human obesity and diabetes". Nutrition & Diabetes. 4 (9): e135. doi:10.1038/nutd.2014.30. PMC 4183971
. PMID 25198237.
- ↑ Andrikopoulos, Sofianos; Massa, Christine M.; Aston-Mourney, Kathryn; Funkat, Alexandra; Fam, Barbara C.; Hull, Rebecca L.; Kahn, Steven E.; Proietto, Joseph (2005-10-01). "Differential effect of inbred mouse strain (C57BL/6, DBA/2, 129T2) on insulin secretory function in response to a high fat diet". Journal of Endocrinology. 187 (1): 45–53. doi:10.1677/joe.1.06333. ISSN 0022-0795. PMID 16214940.
- ↑ Fearnside, Jane F.; Dumas, Marc-Emmanuel; Rothwell, Alice R.; Wilder, Steven P.; Cloarec, Olivier; Toye, Ayo; Blancher, Christine; Holmes, Elaine; Tatoud, Roger (2008-02-27). "Phylometabonomic Patterns of Adaptation to High Fat Diet Feeding in Inbred Mice". PLOS ONE. 3 (2): e1668. doi:10.1371/journal.pone.0001668. ISSN 1932-6203. PMC 2244706
. PMID 18301746.
- ↑ Chandrasekera, P. Charukeshi; Pippin, John J. (2014-01-01). "Of rodents and men: species-specific glucose regulation and type 2 diabetes research". ALTEX. 31 (2): 157–176. ISSN 1868-596X. PMID 24270692.
- ↑ Gajda, Angela M. "How to made hight fat diet?". www.researchgate.net. Research Diets inc. Retrieved 14 November 2016.
- ↑ Gadja, Angela M.; Pellizzon, Michael A.; Ricci, Matthew R.; Ulman, Edward A (30 April 2008). "Diet-Induced Metabolic Syndrome in Rodent Models". ALN. Advantage Business Media. Retrieved 14 November 2016.
- ↑ Conn, P. Michael (2013-05-29). Animal Models for the Study of Human Disease. Academic Press. ISBN 9780124159129.
- ↑ Harwood, H James; Listrani, Paul; Wagner, Janice D (2012-05-01). "Nonhuman Primates and Other Animal Models in Diabetes Research". Journal of Diabetes Science and Technology. 6 (3): 503–514. ISSN 1932-2968. PMC 3440045
. PMID 22768880.
- ↑ Balcombe, J. P. (2006-07-01). "Laboratory environments and rodents' behavioural needs: a review". Laboratory Animals. 40 (3): 217–235. doi:10.1258/002367706777611488. ISSN 0023-6772. PMID 16803640.
- 1 2 Eckel-Mahan, Kristin; Sassone-Corsi, Paolo (2016-11-13). "Metabolism and the Circadian Clock Converge". Physiological Reviews. 93 (1): 107–135. doi:10.1152/physrev.00016.2012. ISSN 0031-9333. PMC 3781773
. PMID 23303907.
- ↑ King, Aileen JF (2016-11-13). "The use of animal models in diabetes research". British Journal of Pharmacology. 166 (3): 877–894. doi:10.1111/j.1476-5381.2012.01911.x. ISSN 0007-1188. PMC 3417415
. PMID 22352879.
- ↑ Spencer, Geoff. "Background on Mouse as a Model Organism". Genome.gov. National Human Genome Research Institute. Retrieved November 13, 2016.
- ↑ Upadhyay, Vaibhav; Poroyko, Valeriy; Kim, Tae-jin; Devkota, Suzanne; Fu, Sherry; Liu, Donald; Tumanov, Alexei; Koroleva, Ekaterina; Deng, Liufu (26 August 2012). "Lymphotoxin regulates commensal responses to enable diet-induced obesity". Nature Immunology. 13 (10): 947–953. Retrieved 7 November 2016.
- ↑ Kohei, Jobu; Junko, Yokota; Saburo, Yoshioka; Hironori, Moriyama; Shuzo, Murata; Masao, Ohishi; Hiroyuki, Ukeda; Mitsuhiko, Miyamura (November 2013). "Effects of Goishi tea on diet-induced obesity in mice". Food Research International. 54 (1): 324–329. Retrieved November 7, 2016.
- ↑ Koya-Miyata, Satomi; Arai, Norie; Mizote, Akiko; Taniguchi, Yoshifumi; Ushio, Shimpei; Iwaki, Kanso; Fukuda, Shigeharu (December 2009). "Propolis Prevents Diet-Induced Hyperlipidemia and Mitigates Weight Gain in Diet-Induced Obesity in Mice". Biological and Pharmaceutical Bulletin. 32 (12): 2022–2028. Retrieved November 7, 2016.
- ↑ Gao, W.R.; Zhu, W.L.; Zhou, Q.H.; Zhang, H.; Wang, Z.K. (27 May 2014). "Diet induced obesity in Apodemus chevrieri". Italian Journal of Zoology. 81 (2): 235–245. Retrieved November 7, 2016.
- ↑ "Selective breeding for diet-induced obesity and resistance in Sprague-Dawley rats". American Journal of Physiology. 273.
- ↑ "Propensity to high-fat diet-induced obesity in rats is associated with changes in the gut microbiota and gut inflammation". American Journal of Physiology. 299.
- ↑ "Dogs". Office of Research Integrity. Retrieved Nov 14, 2016.
- ↑ "Diet-Induced Obesity Prevents Interstitial Dispersion of Insulin in Skeletal Muscle". Diabetes. 59.
- 1 2 Bray, George A.; Paeratakul, Sahasporn; Popkin, Barry M. (2004-12-30). "Dietary fat and obesity: a review of animal, clinical and epidemiological studies". Physiology & Behavior. Dietary Fat and Energy Balance-Myths and Facts. 83 (4): 549–555. doi:10.1016/j.physbeh.2004.08.039.
- 1 2 3 4 Hariri, Niloofar; Thibault, Louise (2010-12-01). "High-fat diet-induced obesity in animal models". Nutrition Research Reviews. 23 (2): 270–299. doi:10.1017/S0954422410000168. ISSN 1475-2700.